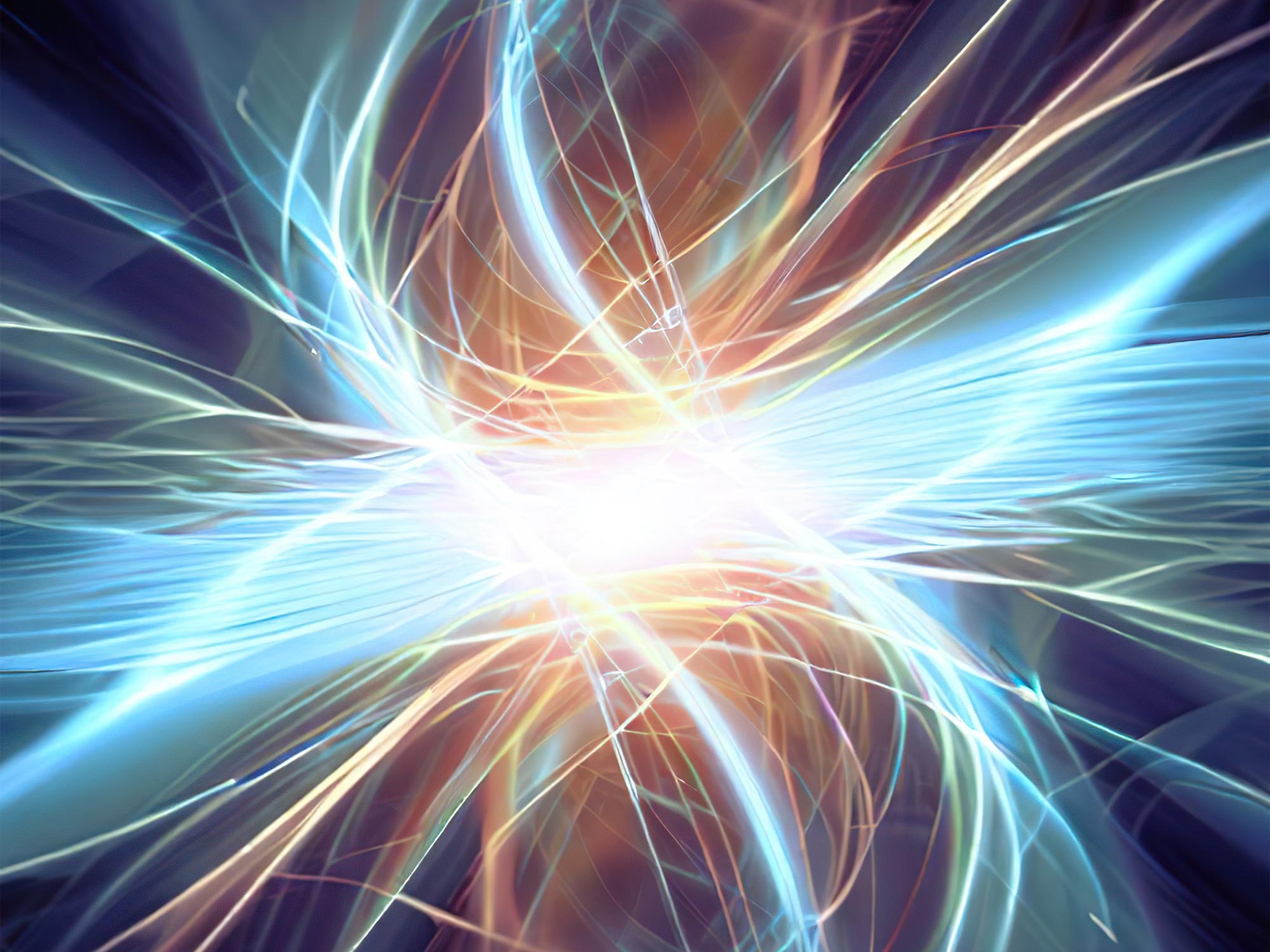
اكتشف علماء فيزياء ولاية بنسلفانيا تفاعلًا عالميًا في الأنظمة الكمومية عندما تتعطل بسبب تدفق كبير للطاقة. باستخدام الغازات شديدة البرودة أحادية البعد ، تمكنوا من مراقبة هذه الاستجابة عن كثب والمرحلة اللاحقة المعروفة باسم “الديناميكا المائية” ، مما وفر نموذجًا لفهم أنظمة الكم المماثلة. تم نشر النتائج في المجلة طبيعة.
ألقت التجارب الجديدة مع الغازات الذرية شديدة البرودة الضوء على كيفية تطور جميع الأنظمة الكمومية المتفاعلة بعد التدفق المفاجئ للطاقة.
تكشف التجارب الجديدة التي تستخدم غازات أحادية البعد من الذرات شديدة البرودة عن عالمية في كيفية تغير الأنظمة الكمومية المكونة من العديد من الجسيمات بمرور الوقت بعد تدفق كبير للطاقة يؤدي إلى عدم توازن النظام. أظهر فريق من الفيزيائيين من ولاية بنسلفانيا أن هذه الغازات تتفاعل على الفور ، و “تتطور” مع خصائص مشتركة لجميع أنظمة “الأجسام المتعددة” الكمومية وبالتالي غير متوازنة. نُشر مقال يصف التجارب في 17 مايو 2023 في المجلة طبيعة.
قال ديفيد فايس ، أستاذ الفيزياء الفخري في ولاية بنسلفانيا وأحد قادة فريق البحث: “العديد من التطورات الرئيسية في الفيزياء خلال القرن الماضي كانت تتعلق بسلوك العديد من الجسيمات الكمومية.” “على الرغم من المجموعة الرائعة من ظواهر” العديد من الأجسام “المختلفة ، مثل الموصلية الفائقة والسائل الفائق والمغناطيسية ، فقد وجد أن سلوكهم بالقرب من التوازن غالبًا ما يكون متشابهًا بدرجة كافية بحيث يمكن تصنيفهم في مجموعة صغيرة من الفئات العالمية. ، لقد أدى سلوك الأنظمة البعيدة عن التوازن إلى ظهور عدد قليل من هذه الأوصاف الموحدة.

تجارب جديدة مع الغازات الذرية شديدة البرودة تكشف عن فيزياء عالمية في ديناميكيات الأنظمة الكمومية. يقف يوان لي ، طالبة الدراسات العليا في ولاية بنسلفانيا ، المؤلف الأول للورقة التي تصف التجارب ، بالقرب من الجهاز الذي استخدمته لإنشاء ودراسة غازات أحادية البعد بالقرب من الصفر المطلق. الائتمان: ديفيد فايس ، ولاية بنسلفانيا
وأوضح فايس أن هذه الأنظمة الكمومية متعددة الأجسام عبارة عن مجموعات من الجسيمات ، مثل الذرات ، التي تتمتع بحرية الحركة بالنسبة لبعضها البعض. عندما تكون مزيجًا كثيفًا وباردًا بدرجة كافية ، والتي يمكن أن تختلف اعتمادًا على السياق ، فإن ميكانيكا الكم – النظرية الأساسية التي تصف خصائص الطبيعة على النطاق الذري أو دون الذري – ضرورية لوصف ديناميكياتها.
يتم إنشاء أنظمة خارج التوازن بشكل كبير بانتظام في مسرعات الجسيمات عندما تصطدم أزواج من الأيونات الثقيلة بسرعات تقترب من سرعة الضوء. ينتج عن الاصطدامات أ[{” attribute=””>plasma—composed of the subatomic particles “quarks” and “gluons”—that emerges very early in the collision and can be described by a hydrodynamic theory—similar to the classical theory used to describe airflow or other moving fluids—well before the plasma reaches local thermal equilibrium. But what happens in the astonishingly short time before hydrodynamic theory can be used?
“The physical process that occurs before hydrodynamics can be used has been called ‘hydrodynamization,” said Marcos Rigol, professor of physics at Penn State and another leader of the research team. “Many theories have been developed to try to understand hydrodynamization in these collisions, but the situation is quite complicated and it is not possible to actually observe it as it happens in the particle accelerator experiments. Using cold atoms, we can observe what is happening during hydrodynamization.”
The Penn State researchers took advantage of two special features of one-dimensional gases, which are trapped and cooled to near absolute zero by lasers, in order to understand the evolution of the system after it is thrown of out of equilibrium, but before hydrodynamics can be applied. The first feature is experimental. Interactions in the experiment can be suddenly turned off at any point following the influx of energy, so the evolution of the system can be directly observed and measured. Specifically, they observed the time-evolution of one-dimensional momentum distributions after the sudden quench in energy.
“Ultra-cold atoms in traps made from lasers allow for such exquisite control and measurement that they can really shed light on many-body physics,” said Weiss. “It is amazing that the same basic physics that characterize relativistic heavy ion collisions, some of the most energetic collisions ever made in a lab, also show up in the much less energetic collisions we make in our lab.”
The second feature is theoretical. A collection of particles that interact with each other in a complicated way can be described as a collection of “quasiparticles” whose mutual interactions are much simpler. Unlike in most systems, the quasiparticle description of one-dimensional gases is mathematically exact. It allows for a very clear description of why energy is rapidly redistributed across the system after it is thrown out of equilibrium.
“Known laws of physics, including conservation laws, in these one-dimensional gases imply that a hydrodynamic description will be accurate once this initial evolution plays out,” said Rigol. “The experiment shows that this occurs before local equilibrium is reached. The experiment and theory together, therefore, provide a model example of hydrodynamization. Since hydrodynamization happens so fast, the underlying understanding in terms of quasi-particles can be applied to any many-body quantum system to which a very large amount of energy is added.”
Reference: “Observation of hydrodynamization and local prethermalization in 1D Bose gases” by Yuan Le, Yicheng Zhang, Sarang Gopalakrishnan, Marcos Rigol and David S. Weiss, 17 May 2023, Nature.
DOI: 10.1038/s41586-023-05979-9
In addition to Weiss and Rigol, the research team at Penn State includes Yuan Le, Yicheng Zhang, and Sarang Gopalakrishnan. The research was funded by the U.S. National Science Foundation. Computations were carried out at the Penn State Institute for Computational and Data Sciences.